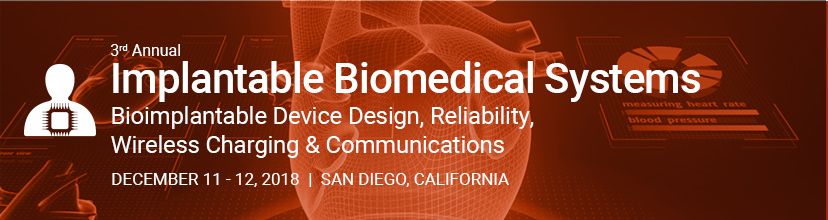
The market demand for implantable biomedical systems continues to grow at a significant pace. Solving the technological hurdles of miniaturization, power supply and efficient interfaces between the implants and external devices is critical to the growth
of the market. This symposium will examine the latest advances in design, manufacturing, reliability, wireless charging and communications for implantable biomedical systems from both a theoretical and practical standpoint.
Final Agenda
Tuesday, December 11, 2018
12:15 pm Registration
1:55 Chairperson’s Remarks
Mark Buccini, Director, Business Unit Strategy, Texas Instruments
2:00 OPENING KEYNOTE PRESENTATION: Enabling New Applications with Multivariable Chem/Bio Sensors: From Ideas to Product
Radislav A. Potyrailo, PhD, Material Characterization & Chemical Sensing, GE Global Research Center
Modern monitoring scenarios of gases and liquids for industrial safety, environmental surveillance, medical diagnostics, and other applications demand sensing with higher accuracy, enhanced stability, and lower power consumption; often all in unobtrusive
formats and at low cost. We are developing a new generation of sensors that bridge the gap between existing and required capabilities. Our methodology allows quantitation of individual chemical or biological components in mixtures, rejection of interferences,
and correction for environmental instabilities.
2:30 Facilitating Collaboration to Advance the Commercialization of Nanosensors: The NNI Sensors Signature Initiative
Lisa Friedersdorf, Director, National Nanotechnology Coordination Office, National Nanotechnology Initiative
3:00 The Emergence of New Sensing Capabilities from Commercially Available Sensors
Thomas Dawidczyk, Lead Analyst, Lux Research
3:30 Coffee Break in the Exhibit Hall with Poster Viewing
4:00 Addressing the Unique Problems in Power Storage for Miniaturized Sensors
Erik Scott, PhD, Bakken Fellow, Technical Fellow, Director of Advanced Development, Medtronic
Successful implementation of miniaturized sensors demands that the power source not only maximizes power and energy density, but also exhibits robust performance over stressful use conditions and long product lifetimes. This entails three cell-level attributes:
ability to recharge quickly; stable performance over many cycles and years; and robustness to extremes in voltage associated with overcharge and over-discharge. Characterization data of Medtronic’s OverdriveTM battery technology will be presented.
4:30 PANEL DISCUSSION: Expanding Implant Applications to Improve Outcomes for Future Applications
Moderator: Bill von Novak, Principal Engineer, Qualcomm
Panelists: Erik Scott, PhD, Bakken Fellow, Technical Fellow, Director of Advanced Development, Medtronic
Reza Sehdehi, Auckland Bioengineering Institute, The University of Auckland, New Zealand
Farah Laiwalla, MD, PhD, Senior Research Associate, Neuroengineering and Nanophotonics Laboratory, Brown University
Deborah Munro, PhD, Adjunct Faculty, OHSU Department of Orthopaedics & Rehabilitation, Oregon Health and Science University; Biomedical Engineering Research Consultant, Munro Medical, LLC
This panel of experts will discuss what missing technology is keeping implants from seeing a wider application space. Also, how medical implants can improve outcomes for currently underserved populations, what is beyond medical implants and what implants
can do beyond life support, therapeutic or palliative applications will be discussed.
5:30 End of Day
Wednesday, December 12, 2018
8:30 am Morning Coffee
8:55 Chairperson’s Opening Remarks
Erik Scott, PhD, Bakken Fellow, Technical Fellow, Director of Advanced Development, Medtronic
9:00 Sources of Energy for Medical Implants
Bill von Novak, Principal Engineer, Qualcomm
Medical implants need energy to operate - from nanowatts (cardiac rhythm management) to tens of watts (ventricular assist devices). Historically, this energy has come from either primary batteries, rechargeable batteries with wireless charging,
or a wired power source. However, there are several other sources of energy available for medical implants, many of which are already present within the human body. This talk will describe some of those sources and how to harvest them for
use in medical devices.
9:30 Effects of Conductive Tissue on Capacitive Wireless Power Transfer
Reza Sehdehi, Auckland Bioengineering Institute, The University of Auckland, New Zealand
Biomedical devices are one of the most successful applications of Inductive Power Transfer (IPT) where it provides a lifetime of power without the risk of infection associated with percutaneous leads. In this study, it was found that the tissue
medium is dominated by its conductive properties at low frequency. In the future, the model and the test phantom will be utilized to understand the implementation and safety challenges in applying the capacitive power technology for powering
implantable devices.
10:00 Coffee Break in the Exhibit Hall with Poster Viewing
10:45 Radiofrequency Ablation with a Wirelessly Powered Catheter and Generator
Julian Moore, UGA Medical Robotics Lab, The University of Georgia
Radiofrequency ablation (RFA) is a minimally invasive medical procedure where tissue is burned in the body with electrical current. Current methods of RFA use several cords and wires that complicate the procedure and present the risk of cutting
or shorting the circuit if they are damaged. This method streamlines the ablation procedure by reducing setup time and eliminating the need for those wires and chords that get in the way of the procedure. The wireless method also has an increased
benefit to sterilization because the wireless needles are disposable and cheaper than the current catheters.
11:15 Wireless Power Transfer for Cardiovascular Implants
Paul Mitcheson, PhD, Faculty of Engineering, Department of Electrical and Electronic Engineering, Imperial College London
Many types of cardiovascular implants such as loop recorders, implantable cardioverter-defibrillators (ICDs) and left ventricular assist devices (LVADs) require electrical power that is normally provided by implantable batteries or a driveline
through an incision. The Wireless Power Lab is collaborating with the National Heart and Lung Institute at Imperial College London to develop wireless powering technology for next-generation cardiovascular implants. This talk focuses on the
various engineering challenges, safety considerations and regulatory issues involved with integrating inductive power transfer into such implants and provides results from an initial feasibility study.
11:45 Miniaturized Hermetic Modules with Integrated Sensors and Communication for Implants
Eckardt Bihler, PhD, Business Development Manager, Dyconex, Switzerland
Liquid Crystal Polymer (LCP), a thermoplastic dielectric material with superior material properties can be used both as the substrate material and as the encapsulation for small miniaturized implantable electronic and modules. Very small form
factors can be obtained by integrating the communication coils into the LCP substrate. Encapsulation in LCP provides hermetically sealed and chemically inert miniaturized electronic modules with integrated sensors and electrodes for implanted
medical applications.
12:15 pm Luncheon Presentation (Sponsorship Opportunity Available) or Enjoy Lunch on Your Own
1:40 Chairperson’s Remarks
Bill von Novak, Principal Engineer, Qualcomm
1:45 Circuits and System Design for Implantable Wireless Neural Sensors
Vincent Leung, PhD, Technical Director, Qualcomm Institute Circuits Labs, UCSD
Wireless biomedical implantable systems present unique engineering challenges to RF/analog circuit designers. This talk will start with a survey of circuits and system architectures, where key design parameters and their optimizations will be
explained. We will then focus on a recent research of CMOS distributed wireless sensor IC’s for brain machine interface applications.
2:15 From Wireless Implantable Sensors to an Implant System: A Biomedical Device Development Journey
Farah Laiwalla, MD, PhD, Senior Research Associate, Neuroengineering and Nanophotonics Laboratory, Brown University
An approach for large-scale adaptive sensor systems is critical for scaling biomedical implants beyond a few hundred channels. We describe the development of microscale sensor nodes, in the context of development of an adaptive wireless implant
system that enables real-time networked access to thousands of nodes.
2:45 Incorporating Drug Eluting Features to Improve the Performance of Implantable Devices
James Arps, PhD, Director, Business Development, ProMed Pharma, LLC
The human body’s response to an implantable sensor or device can be unexpected and sometimes deleterious. The potential for infection, allergic reaction, or inflammatory response must be considered in design of the next generation of implantable
systems. This talk will review approaches to delivery of therapeutic agents to mitigate such effects including formulations and designs to achieve optimal performance.
3:15 Networking Refreshment Break
3:30 Thin Film 3-D Hermetic Packaging of Sub-mm Wireless Microelectronic Sensor/Actuator Implants
Joonso Jeong, PhD, Assistant Professor, Pusan National University, Korea
Effective 3-dimensional hermetic encapsulation of sub-mm sized implantable microelectronic chiplets is demonstrated by thin (100 nm) multilayer dielectric HfO2/SiO2 atomic layer deposited coatings. Accelerated aging tests at 87 C in saline of
recently developed wireless microchips for neural sensing devices predict many years of chronic implant utility as medical sensor implants.
4:00 Fabricating Implantable MEMS Sensors in Open Use Labs
Deborah Munro, PhD, Adjunct Faculty, OHSU Department of Orthopaedics & Rehabilitation, Oregon Health and Science University; Biomedical Engineering Research Consultant, Munro Medical, LLC
One of the major objectives of the National Nanotechnology Initiative (NNI), established in 2000, was to create user facilities and networks that were available to all. To alleviate this discrepancy for both smaller academic institutions and commercial
enterprises, the NSF developed a new model of support known as NNCI. This presentation covers how to access and collaborate with one of the 16 open facilities located across the United States for fabricating your own biomedical implantable
MEMS sensors and actuators at a reasonable cost.
4:30 End of Implantable Biomedical Systems